Project Profile
This project was cooperated with Shanfei Zhang, a Ph.D. Student supervised by Prof. Bin Su. This project aims to achieve a 4D-printing magnetoelectric soft actuator with ultra-fast response and ultra-low sensing limit. And I was responsible for part of the experiments (design, fabrication, etc) and most of the simulation (COMSOL). Here, I would like to briefly record the simulation by COMSOL.
Figure 1 illustrates the structure of the soft actuator, which consists of a coil, a 3D-printed support structure, and a magnet. Upon energizing the coil, it experiences a downward amperometric force within the magnetic field generated by the magnet. This force prompts rapid deformation of the actuator. Following the cessation of current flow, the actuator reverts to its original shape, driven by the inherent elasticity of the support structure.
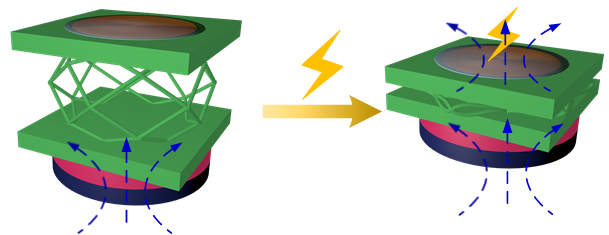
Simultaion Part
The process was simulated using COMSOL software. Initially, the 4D-printed material underwent testing to ascertain its material properties, which include a Young’s modulus of 1.235 MPa, a density of 1050 kg/m^3, and a Poisson’s ratio of 0.3. In the simulation setup, both the coil and the magnet were treated as rigid bodies. The magnet exhibited a residual flux density of 1.2 T, while the coil had a diameter of 0.2 mm and consisted of 75 turns.
Next, we conducted simulations to visualize the magnetic field (refer to Figure 2b) generated between the energized coil and the magnet during a primary deformation (refer to Figure 2a). When the coil is inactive, it does not produce a magnetic field, and the surrounding magnetic field is solely generated by the lower magnet. The magnetic field distribution around the soft actuator is depicted in Figure 3b1.
Upon applying a voltage to the coil, a magnetic field forms around it due to the current-induced magnetic effect, as illustrated in Figure 3b2 to Figure 3b4. The magnetic field produced by the energized coil aligns with that generated by the lower magnet, resulting in an attractive amperometric force between the two components. Consequently, the actuator begins to deform in response to this force. The displacement and stress patterns during a compression process are presented in Figure 2c and d, respectively.

The deformation of varied support structures under pressure was simulated by the steady-state solver of the COMSOL structural mechanics module and the reaction forces are calculated for guiding and interpreting the actual experiments. The stress distributions of vatied structures under the same displacement are shown in Figure 3. By combining experimental and simulation results, we can select the most suitable structure for the soft actuator.

Brief Conclusion
This work demonstrates a soft actuator based on amperometric force. Moreover, the soft actuator functions as an ultra-sensitive sensor, capitalizing on the principles of electromagnetic induction. It demonstrates the capability to detect the motion of ants and even distinguish between varying frequencies in the sounds produced by a tuning fork. This work has been completed and the manuscript is being prepared.